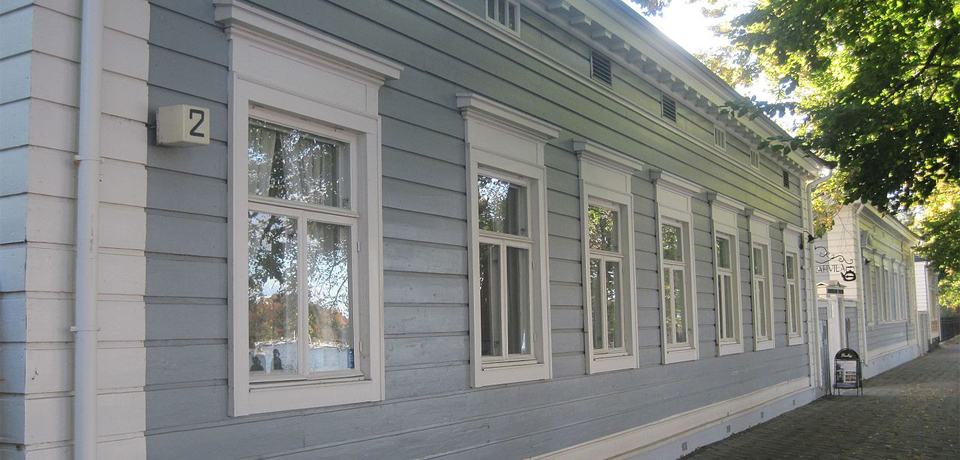
Roosa Helander, Juha Jordan & Päivi Laaksonen
Bio-based colorants are a potential way of increasing the sustainability of consumer products and the construction industry. Potential feasible sources for bio-based dyes and pigments include for instance, agricultural production of biomass and side streams of industries. This paper presents a study on the suitability of indigo pigment in a fully bio-based coating intended for the protection of wooden facades.
Introduction
Demand for sustainable colorants
Today’s mindset for sustainability and circular economy is based on the need to create and utilize products and manufacturing methods that do not harm the environment. Research and development of various bio-based raw materials have increased, and alternatives to fossil-based chemicals and materials are constantly being sought. The current dyeing industry uses mostly synthetic dyes. In 2019, the global dyeing industry market size was valued at 33.2 billion USD (Grand view research, 2020), and the share of natural dyes in the global market in 2019 was instead 6.76 billion USD, including also the controversial azo dyes. (Reports and data, 2020). The use of dyes made from natural compounds is still rather rare on an industrial scale, and even bio-based materials must be dyed with synthetic dyes in the absence of alternatives. Therefore, sustainable dyes must be developed to meet the needs of the dyeing industry, in line with the principles of nature and sustainability.
Natural colorants obtained from plants, insects, animals and various soils have almost always been utilized in human history. Synthetic dyes superseded natural dyes in the 20th century and still dominate the dye industry despite their adverse effects on nature, animals and humans. (Cardon, 2010) Traditionally, natural colors are used mostly for fabric dyeing, such as cotton, wool, silk and linen. (Gupta, 2019) However, for example, wood, paper and leather can also be dyed with natural colorants. (Bechtold & Mussak, 2009; Sivakumar et al., 2009) In this paper, we study the potential of natural indigo pigment in a fully bio-based coating intended for wooded facades. (Helander, 2020)
Natural Indigo is produced in plants
Stable blue colorants are rarely found in nature. Most of the blue colorants in large-scale use are either naturally occurring or synthetically obtained mineral pigments, such as ultramarine, azurite, cobalt blue and Prussian blue. (Pro Puu -yhdistys, 2023) All of these are commercially available, but they all come from non-renewable sources, and some of them are regarded as semi-precious gemstones, meaning that their abundance is low and recovery expensive and resource intensive. Therefore, we turn our interest to the most stable blue pigment obtained from plant biomass, the natural indigo and its potential for the coloration of wood coatings. Indigo has also been found in historical blue pigments including Maya Blue, which is a hybrid of organic indigo and minerals (Berke, 2007).
Indigo is a deep blue pigment that can be found in some plants, but it can also be produced synthetically from the by-products of the oil and coal tar industry. The chemical structure of synthetic and natural indigo is the same (Figure 1A) (Stoker et al., 1998), but the color and properties of natural indigo and synthetic indigo differ due to the impurities that the natural indigo contains. (Balfour-Paul, 2006, pp. 83–87) Among the natural colorants, indigo has a remarkably high photostability, which makes it a potential colorant for many uses. (Yamazaki et al., 2011) Indigo is a water-insoluble molecule, and for textile dyeing, a vat process, including a step of chemical reduction converging the pigment into a soluble form, is needed before the dye is fixed on the garment upon oxidation. However, for the coloration of polymeric materials such as paints and plastics, the insoluble indigo pigment can be readily utilized. (Jordan et al., 2021)
Indigo is found in plants as colorless precursors, indican and isatan B. There are dozens of plants containing these molecules, most of which grow in Asia. Indigo-containing plants growing in Europe are woad (Isatis tinctoria) and Japanese Indigo (Persicaria tinctoria). (Tumer et al., 2017) The agricultural production of indigo is carried out by growing indigo plants and woad (Figure 1B–C). In Europe, indigo is mainly produced in the leaves of woad which is a biennial plant that in first year of growth only produces leaves and blooms, and seeds in the second year of growth. The extraction process of indigo is generally similar for different production plants and is described in Figure 1D. The water-soluble substances are extracted from the plant parts to warm water. Extraction should take place as soon as possible after leaf collection as the yield of indigo pigment decreases over time from the moment of leaf collection (Roberts, 2020). Warm water releases enzymes from the plant leaves, which in turn release indoxine radicals from water-soluble indigo precursors isatan B and indican. Indoxyl radicals form a yellow-greenish leuco-indigo, which can be oxidized to blue indigo, that precipitates from the solution. (Stoker et al., 1998) The oxidation process is accelerated by aerating the indigo broth. The whole extraction process can be accelerated by alkaline conditions. If the leaves receive oxygen during extraction, indoxyl radicals may form isatin, which causes the formation of side products such as red indirubin. (Bechtold & Mussak, 2009)
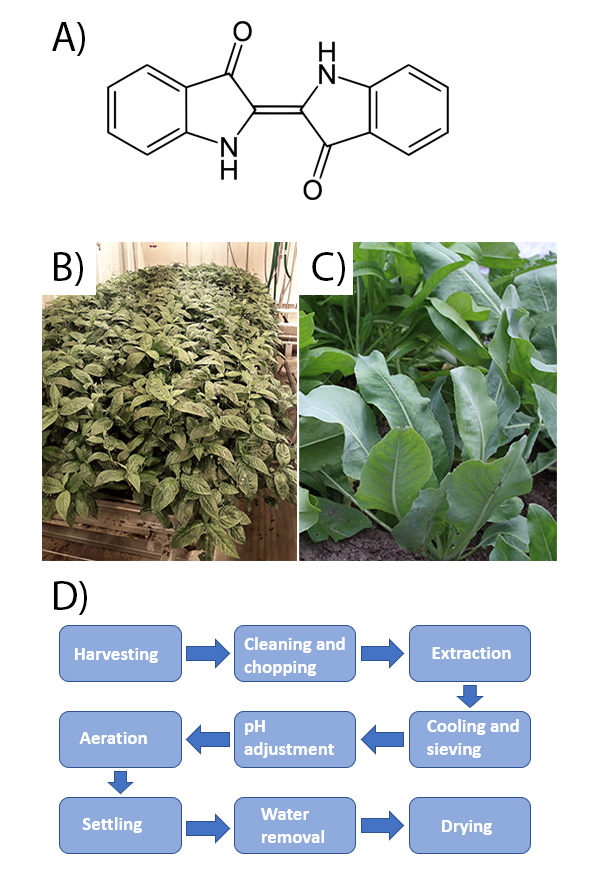
This study focused on the applicability of natural indigo in a coating of construction materials, which is one of the potential application areas for natural dyes and pigments. Especially protective and aesthetic coatings of wooden materials indoors and outdoors are of interest. The demands for the stability of the colorants vary according to the exposure to climatic conditions such as humidity, sunlight and temperature, and there are standardized methods for assessment of these properties. In this work, accelerated weathering was applied to evaluate the stability of natural indigo in conditions simulating long-term exposure to outdoor conditions. The purpose of these experiments was to understand the suitability of natural indigo as a colorant for wooden facades and also to identify its potential limitations that may narrow down the applicability to indoor applications. The applied accelerated climate test could reproduce in a few weeks’ time period, the stress that would require a period of years outdoors. As the experiment progressed, changes in color and gloss of the coatings were monitored to provide information on the durability and protective performance of coatings.
Materials and Methods
Sample preparation
The purpose of this study was to determine the stability of indigo pigments mixed with traditional linen oil (Uula Linseed Oil Paint, Uula Color Oy) applied as a coating on wood. A comparison between natural indigo (Natural Indigo Finland), synthetic indigo (Dye content 95 %, Sigma-Aldrich), and a commercial inorganic blue pigment (Blue Pigment 605, Uula Color Oy) was carried out. This inorganic pigment was chosen as a reference because it is a commonly used pigment known to have good lightfastness properties. The coating composition was prepared by mixing five percent of each pigment powder with linen oil. Planed and sanded pine board was used as a sample material in the work. Four layers of the pigmented linen oils were applied on the sample surface using a brush. An uncoated surface and unpigmented linen oil were used as the reference samples.
Accelerated weathering
The effect of UV light, temperature and humidity were investigated using an accelerated weathering test (QUV Accelerated Weather Tester, QUV/Spray, Q-Lab) (Figure 2A). The effects of this test on the samples were monitored by both color and gloss measurements of the sample surfaces. The total length of the test period was 12 weeks, and the measurements were performed weekly. The operation of the device is based on alternating cycles of UV radiation and condensation, which simulates natural sunlight, rain, and dew. The test cycle consisted of continuous exposure over four-hour periods to alternating UV radiation at 60 degrees Celsius and condensation humidity at 40 degrees Celsius (Figure 2B).
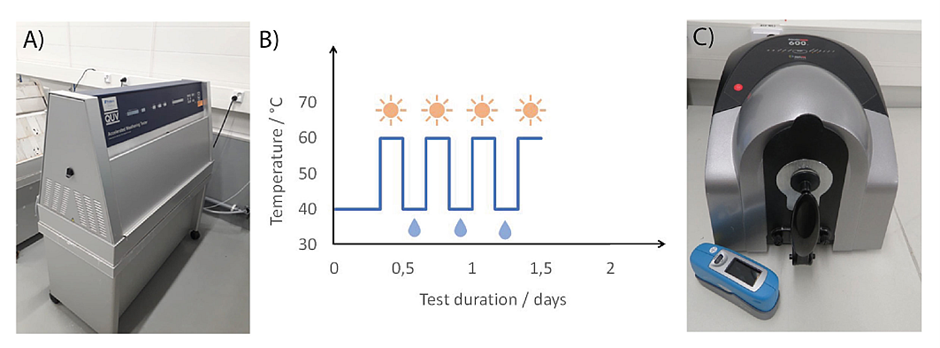
The accelerated laboratory weathering test provides valuable data because it allows the experiments to be controlled and repeated if necessary. In natural weathering tests, the studied samples are exposed to very complex climatic conditions for a long time period, which, on one hand, gives a more reliable result than the laboratory tests, but on the other hand, has a considerably longer duration and variation that may be non-repeatable. Outdoor testing is often done for products or prototypes, that have already been identified in other tests as viable candidates for production. Accelerated tests are suitable for the research phase of new products to avoid using excessive time for examining samples completely unsuitable for outdoor use because a rough estimate of the weather resistance of the sample is obtained fast. Because it is not artificially possible to fully simulate the natural decomposition of wood under outdoor conditions, tests tend to focus on monitoring changes caused by UV light, humidity, and temperature. The same factors that make up the weather cause the biodegradation of the wood and have a detrimental effect on the protective treatment of the wood, such as in this case, coatings. (Teacă et al., 2013)
Analysis of the color and gloss changes due to UV exposure
Color measurements of the coated wood samples were performed by measuring the reflection spectra of the sample surface before and after each exposure cycle (Datacolor 600TM spectrophotometer, Datacolor) (Figure 2C). Color determination was based on the CIELAB system, which analyses colors based on coordinates representing opposing colors. The CIELAB coordinates include color brightness L*, red/green coordinate a*, and yellow/blue coordinate b*. The coordinates are based on the color-opponent theory stating that color cannot be red and green or yellow and blue at the same time and is able to address each color with a unique combination of coordinates. The measurement of L* value indicates the brightness of the color between 0 and 100, where 0 means completely black and 100 means completely white. L* is also called luminance or lightness. The a* and b* values describe the color itself, typically on a scale from -100 to +100. The L*, a*, and b* values together form a color space, where positive a* values determine the redness of the color and negative values determine the greenness of the color. In turn, positive b* values determine the yellowness of the color and negative blueness. (Lai So et al., 2014) Based on the measured values, the total color change during the sample exposure ΔE can be calculated using equation 1:
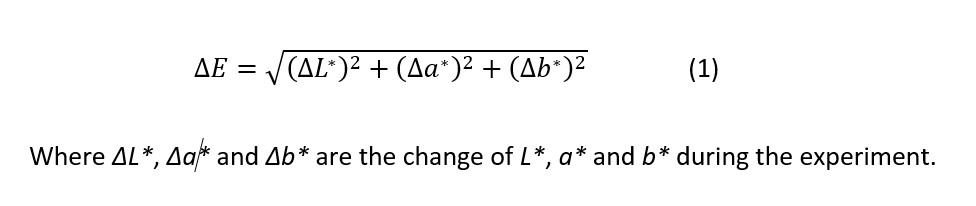
Gloss measurements were performed with a portable gloss meter (micro-TRI-gloss meter, BYK Instruments). The operation of a gloss meter is based on a standardized light source that reflects parallel light to the surface to be measured, as well as a detector that receives light reflected from the surface. Gloss units (GU) are based on a highly polished black reference glass standard with a reflectance of 100 GU at a specified angle. This standard is used to determine the upper point calibration 100, with the lower endpoint set to 0 on a fully matte surface. This scaling is applicable to most coatings and materials, such as paints and plastics, as they generally fall into this range. (Rhopoint Instruments, 2020)
Results and discussion
Changes in color
The color and gloss of the samples after each exposure cycle are shown in Figure 3. The brightness L of all the studied samples decreased due to the weathering (Figure 3A). (Helander, 2020) Most of the changes occurred during the first 5 exposure cycles after which the L stabilized at certain values. The largest changes were obtained for the uncoated wood and unpigmented oil. The pigments that went through the largest loss of brightness were natural indigo and the inorganic pigment, whereas synthetic indigo was very stable throughout the exposure. Change of the color during the exposure is presented in Figure 3B and allows the comparison between color hues of different coatings. All the samples showed an initial change from blue towards yellow, followed by more pronounced reddening as indicated by the arrows in Figure 3B. After a few cycles, many of the samples turned slightly towards blue maintaining the red coordinate. The smallest changes in the color occurred to the samples coated with oil containing synthetic indigo. The sample coated with 4 layers of unpigmented oil did not change greatly in the experiment.
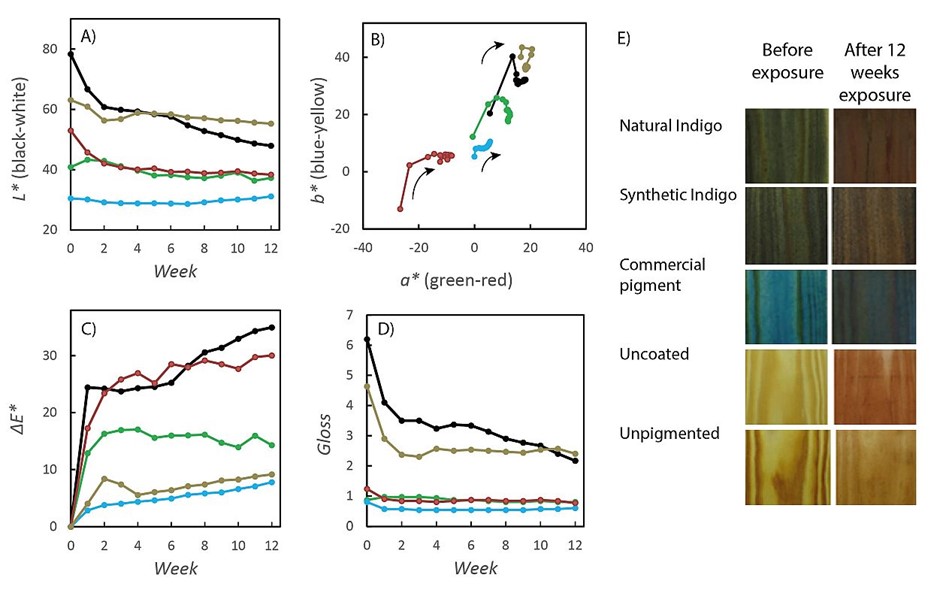
The total color change increased, and the gloss of the samples decreased as a function of the exposure time (Figure 3C–D). (Helander, 2020) For all the samples the major changes occurred during the first 2–3 cycles, after which the values approached a stable value for most of the samples. The uncoated and unpigmented samples, however, stand out in gloss, showing the most unstable properties. An exception was the sample coated with 4 layers of unpigmented oil, which did not change as much as the other unpigmented ones. In total color change, the increase after the initial change was linear for the two unpigmented surfaces and showed no stabilization. In gloss, these two had initially much higher gloss and also went through the largest change compared to the other samples. The samples pigmented with synthetic indigo went through the least change in the total color and the samples containing natural indigo fell in between the two extremes.
Stability of the coatings and wood
When studying the changes in color and gloss, we need to consider the possibility of three changing materials, the wood, the oil and the pigment. As stated in previously published research on weathering of wood that was coated only with non-pigmented wood oil, decreasing of the brightness of bare wood was observed, while the a, b and ΔE values increased with increasing exposure time (Teacă et al., 2013). The rate of color change was also found to be greatest at the beginning of the test as was in the present work. The study of Teacă et al. (2013) stated that the total color change was related to the formation of carbonyl groups, as well as the decomposition of lignin on the surface of the wood. Yellowing of the wood surface was found to reflect changes in lignin and hemicellulose molecules. Similar trends in the color of uncoated wood, as well as coated surface, were obtained in this work (Figure 3), which implies that the color changes of the samples coated with translucent linen oil are largely due to the color changes of the wood rather than the color changes of the pigment.
According to the measurements of the color change (Figure 3C), four layers of the linen oil coating alone appeared to prevent the color change of wood in an effective manner. This implies that the color changes occurring in the wood did not significantly affect the color of the samples. In the comparison of the samples, the synthetic indigo did not significantly change color, whereas the natural indigo and commercial pigment did. Thus, the exposure to UVA radiation, elevated temperature and moisture appeared to damage the natural indigo pigment, whereas the synthetic pure compound was very stable. The possible reasons for the instability of natural indigo are most likely the impurities, such as indigo precursors and residual metals it contains, which can react further and cause changes in the indigo structure. The reason for the poor protective performance of the commercial blue pigment may be that it is not necessarily intended for use in admixture with wood oil.
The gloss measurement showed that all the surfaces fell in the range of low gloss (GU < 10), and the lower the gloss the more stable a property it was. The unpigmented oils and the uncoated wood surface appeared to have initially higher gloss, but they soon decreased during the exposure. Gloss is highly dependent on the surface structure and smoothness, and all the used pigments appeared to create a matte coating, probably due to the roughness created by the pigment particles. The lowering of gloss was a consequence of changes in the top layer of the linen oil coating due to weathering.
In addition to the linen oil coating, it is also important to consider that the pigments may play a role in the protection of the wood and prevention of the changes due to UV radiation. The pigments are chromophores and absorb light and UV radiation effectively. Thus, they can prevent the penetration of UV radiation on the wood surface and the consecutive chemical and structural degradation of its constituents. When comparing the observed color changes, the synthetically produced pure indigo stands out. The coating including synthetic indigo appears not to go through the last steps of color change along the b* axis which is typical for all the other samples. The prevention of the color changes in wood, especially the discoloration that eventually happens is needed for enabling long lasting solutions, for building and construction. For protection of wood, several types of UV absorbing organic compounds are used and many are in the development. (Hayoz et al., 2003) According to our results, the strongly colored indigo blue was able to scavenge UV radiation and thus prevent degradation of the wood surface.
Conclusions
When exposed to UV light, moisture and heat, changes occurred on the wood, the linen oil and the pigment, and identifying and separating these changes were not straightforward. Based on the results of the color change and gloss measurements, the darkening of the wood due to the changes in its chemical structures under the influence of UV radiation, was visible through the coatings. However, applying a thick enough coating layer enabled observation of the difference between the color stability of the pigments and wood. The highest stability was obtained for pure indigo compound, the second highest for the natural indigo, and the least stability for the inorganic pigment that was studied as a reference and example of a commercially available pigment. The experiments also revealed the ability of the synthetic indigo to protect wood against UV radiation, which was probably due to its strong absorbance of light and UV radiation.
The obtained results encourage further research on the bio-based colorants and their functionalities. The purpose of these experiments was to understand the suitability of natural indigo as a dye in wood coatings and whether natural indigo has the potential to function as a dye for outdoor use, or whether its potential use is limited to indoor use only. Based on the results, more detailed research is still needed in such an application where it is possible to exclude the base material’s own changes for example in terms of color and gloss. The experimental designs that enable studying the stability of the pigments and dyes alone will be developed, for instance by mixing the pigment powders with more opaque coating material, such as white paint instead of transparent oil. On the other hand, the opaque coatings and other ways to prevent discoloration of wood are much used and this study also showed potential to maintain color under harsh conditions using organic bio-based colorants.
Authors
Roosa Helander works as a Project Engineer at HAMK Tech and has been working in Bio Based Dyes and Pigments for Colour Palette (BioColour) and Bio-based raw materials value chain (BIO-OSAKE) projects.
Juha Jordan works as a Researcher at HAMK Tech and is working on his doctoral thesis in Bio Based Dyes and Pigments for Colour Palette (BioColour) project.
Päivi Laaksonen works as a Principal Research Scientist at HAMK Tech and works in the Bio Based Dyes and Pigments for Colour Palette (BioColour) and Bio-based raw materials value chain (BIO-OSAKE) projects.
References
Bechtold, T., & Mussak, R. (2009). Handbook of natural colorants. Austria: A John Wiley and Sons, Ltd. Publication.
Balfour-Paul, J. (2006). Indigo. Archtype Publications.
Berke H. (2007). The invention of blue and purple pigments in ancient times. Chem Soc Rev. 36, 15–30. https://doi.org/10.1039/B606268G
Cardon, D. (2010). Natural Dyes, Our Global Heritage of Colors. Textile Society of America Symposium Proceedings, 12. https://digitalcommons.unl.edu/tsaconf/12
Grand view Research. (2020). Dyes And Pigments Market Size, Share & Trends Analysis Report By Product (Dyes (Reactive, Vat, Acid, Direct, Disperse), Pigment (Organic, Inorganic)), By Application, By Region, And Segment Forecasts, 2020–2027. https://www.grandviewresearch.com/industry-analysis/dyes-and-pigments-market
Gupta, V. K. (2019). Fundamentals of Natural Dyes and Its Application on Textile Substrates. Chemistry and Technology of Natural and Synthetic Dyes and Pigments. https://www.intechopen.com/books/chemistry-and-technology-of-natural-and-synthetic-dyes-and-pigments/fundamentals-of-natural-dyes-and-its-application-on-textile-substrates
Hayoz, P., Peter, W., & Rogez, D. (2003). A new innovative stabilization method for the protection of natural wood. Progress in Organic Coatings 48, 297–309. https://doi.org/10.1016/S0300-9440(03)00102-4
Helander, R. (2020). Luonnonindigolla ja puuöljyllä pinnoitettujen puukappaleiden värinkesto kiihdytetyssä ilmastorasitustestissä [Bachelor of Engineering Thesis, Häme University of Applied Sciences]. https://urn.fi/URN:NBN:fi:amk-202005128513
Jordan, J., Helander, R., & Laaksonen, P. (2021). Colour stability of wood coatings pigmented with natural indigo from Isatis tinctoria after accelerated weathering. Coloration Technology, 138, 210–218.
Lai So, V. L., He, L. & Xin, J. H. (2014). Bio-inspired colouration on various textile materials using a novel catechol colorant. RSC Advances, 4/2014, 41081–41086. https://doi.org/10.1039/C4RA06004K
Pro Puu -yhdistys. (2023, May, 12). Siniset. PuuProffa. https://puuproffa.fi/puutieto/pintakasittelytavat/pigmentit/siniset/
Reports and data. (2020). Organic Dyes Market By Dyeing Process, By Source (Plant, Animal), And By Application (Agriculture, Textile, Paint & Coatings Industry), Forecasts To 2027. https://www.reportsanddata.com/report-detail/organic-dyes-market
Rhopoint Instruments. (2020). How is gloss measured. https://www.rhopointinstruments.com/faqs/how-is-gloss-measured/
Sivakumar, V., Lakshmi Anna, J., Vijayeeswarri, J., & Swaminathan, G. (2009). Ultrasound assisted enhancement in natural dye extraction from beetroot for industrial applications and natural dyeing of leather. Ultrasonics Sonochemistry, 16(6), 782–789. http://www.sciencedirect.com/science/article/pii/S1350417709000479
Stoker, K. G., Cooke, D. T. & Hill, D. J. (1998). An improved method for the large-scale processing of woad (Isatis tinctoria) for possible commercial production of woad indigo. Journal of Agricultural Engineering Research, 4/1998, 315–320. https://doi.org/10.1006/jaer.1998.0329
Teacă, C.-A., Roşu, D., Bodîrlău, R., & Roşu, L. (2013). Structural Changes in Wood under Artificial UV Light Irradiation Determined by FTIR Spectroscopy and Color Measurements – A Brief Review. Bioresources. 1/2013, 1478–1507. https://ojs.cnr.ncsu.edu/index.php/BioRes/article/view/BioRes_08_1_1478_Teaca_Review_Structural_Changes_Wood_UV/1972
Tumer, T. B., Schmidt, B. M., & Isman, M. (2017). Europe. Ethnobotany. (277–307). https://doi.org/10.1002/9781118961933.ch7
Yamazaki, S., Sobolewski, A. L., & Domcke, W. (2011). Molecular mechanisms of the photostability of indigo. Phys. Chem. Chem. Phys. 13, 1618–1628. https://doi.org/10.1039/C0CP01901A